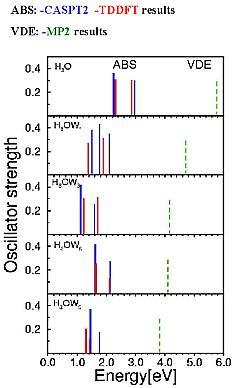 |
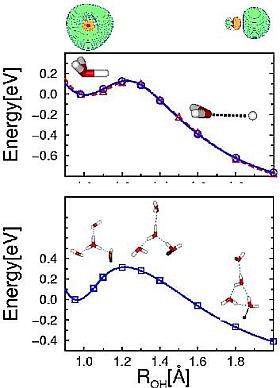 |
Fig.6 Electronic absorption spectra (ABS)
and vertical detachment energy (VDE)
|
Fig.7 Stability of
(n=0,3) clusters
|
Conclusions
radicals can be formed by a barrierless hydrogen-transfer reaction
in the lowest excited singlet state of water clusters or in the lowest
state of an organic chromophore complexed with water.
Microsolvation of the
radical leads to a charge-separated complex,
consisting of a hydronium radical and a localized electron cloud, connected by a water network.
The vertical excitation energies of the s p electronic transitions of the
clusters cover roughly the energy range of the absorption spectrum of the hydrated electron.
Microsolvation with water stabilizes considerably the (unstable)
radical, but for n=3 it is still a metastable species which can decay by detaching the hydrogen atom.
clusters could be the carriers of the characteristic properties of the hydrated electron.
|
References
1. A. L. Sobolewski and W. Domcke, "Photoinduced electron and proton transfer in phenol
and its clusters with water and ammonia",J. Phys. Chem. A 105 (2001) 9275
2. A. L. Sobolewski and W. Domcke, "Hydrated hydronium: a cluster model of the solvated
electron?", Phys. Chem. Chem. Phys., 4 (2002) 4
3. A. L. Sobolewski, W. Domcke, "Photochemistry of
: a cluster model of the
photodetachment of the chloride anion in water", J. Phys. Chem. A, submitted
|