Evolution of Microwave Spectroscopy at the
National Bureau of Standards (NBS) and the National Institute of Standards and
Technology (NIST)
F.J. Lovas
Sensor Science Division, National Institute of
Standards and Technology
Gaithersburg, MD 20899
lovas@nist.gov
D.R. Lide, Jr.
Editor, Electronic Editions, CRC Handbook of Chemistry
and Physics
13901 Riding Loop Dr., North Potomac, MD 20878
R.D. Suenram
4053 Weakley Hollow Rd., Syria, VA 22743
D.R. Johnson
5605 Merlyn Lane, Cape Coral, FL 33914
Abstract
This paper
describes the beginning and evolution of microwave rotational spectroscopic
research starting in 1954 at the National Bureau of Standards (NBS), located at
that time in Washington, DC, through the present at NIST in Gaithersburg, MD.
David Lide was hired in 1954 to start this research employing Stark modulated
waveguide septum cells. When Donald R. Johnson joined the lab in 1968, he
developed parallel plate cells coupled with rf and DC discharge methods to
study free radicals and transient species. In the mid 1980s Lovas and Suenram
constructed a pulsed molecular beam Fourier Transform microwave (FTMW)
spectrometer to study hydrogen bonded and van der Waals dimers and trimers.
This article describes the types of molecules studied and the type molecular
properties derived from these measurements as well as some of the instruments
developed for these studies. The two major areas of application described are
atmospheric chemistry and molecular radio astronomy.
Key words:
atmospheric chemistry, dimers, hydrogen bonding, internal rotation, microwave
spectroscopy, radio astronomy, rotational spectrum, tunneling motions
Introduction
The first work on
microwave spectroscopy at the National Bureau of Standards was carried out in
the late 1940s by Harold Lyons. This was instigated by NBS Director Edward U.
Condon to follow up an idea he got while in his previous position as Director
of the Westinghouse Research Laboratories in East Pittsburgh. Westinghouse Labs
was the scene of the first high-resolution microwave measurements on record,
done by William Good (Goo46) in 1946 on the 23.9 GHz transitions of ammonia.
Condon recognized that resonances of this type in low-pressure gases might be
used as secondary frequency standards, and might even replace the primary
astronomical time/frequency standard in use since the adoption of the Treaty of
the Meter in 1875. Lyons succeeded in 1949 in using an NH3 microwave
line to control a clock. His research showed that the concept was promising,
but Doppler and wall-collision broadening limited the line widths that could be
obtained, and hence the accuracy of the clock. Rapid advances in atomic and
molecular beam measurements soon showed that the beam technique had major
advantages over using bulk gases, and the work on the ammonia frequency
standard was abandoned.
Development of Microwave Spectroscopy
at NBS
NBS began a sustained
research program in microwave spectroscopy in 1954 as a part of a major
thrust
in the fields of thermodynamics and thermophysics. The formulations of
statistical mechanics relating macroscopic thermodynamic properties such
as
heat capacity and entropy to molecular energy levels was well
established, and
this provided a motivation for investigating vibrational and rotational
spectra. The Thermodynamics Section hired David Mann in 1951 to set up
an
infrared spectroscopy program. Mann had worked with David Lide (shown at
right)
in the microwave laboratory of E. B. Wilson at Harvard, and in 1954 he
recruited Lide to join NBS and start a microwave program. Lab space was
obtained and the first equipment ordered in late
1954.
The first spectrometer
was a conventional 80 kHz Stark modulation instrument with a 15 foot X-band
waveguide cell, very similar to the spectrometers in the Harvard lab.
Components that could not be purchased from commercial sources were constructed
at NBS or by contractors. The first problem undertaken, serving as a test of
instrument, was a reinvestigation of sulfuryl fluoride, SO2F2
(Lid57), which cleared up a previous error in the dipole moment and provided
interesting information on the low-lying vibrational states. A strong Coriolis
interaction between the two lowest excited vibrational states was confirmed,
and this permitted a determination of the frequency of one of these states,
which had not been observed directly in the infrared spectrum. In the future
this tool was to be used often to locate vibrational levels that could not be
observed directly.
The microwave program
initially had two thrusts, internal rotation and other low-frequency motions,
and the spectra of transient species. Molecules with internal rotation or
inversion modes were characterized by very low-lying energy levels, which made
large contributions to the thermodynamic properties. Since thermodynamic data were
becoming more important for industrial and military applications, funding could
be obtained fairly easily for this work. There was similar interest in the
molecular species present in high-temperature systems and plasmas. Lide had
first worked on a hindered internal rotation problem while a summer student at
the Westinghouse Labs in 1950, where he studied the spectrum of methyl silane
and was able to estimate the barrier height from intensity measurements on
torsional satellites (Lid50). In 1956 he started measurements on a series of
molecules exhibiting internal rotation, while a new spectrometer designed for
studying free radicals was under construction.
The permanent staff in
the NBS microwave group was limited to David Lide and David Mann during this
period, and Mann was concerned mainly with infrared spectra and administrative
duties. However, the group was fortunate in being able to bring in a large number
of postdoctoral fellows, summer students, and visiting scientists.
This was facilitated by
the establishment of the NBS-NRC Postdoctoral program through the efforts of
David Mann and Joseph Hilsenrath in the mid-1950s. With the help of this
program and others, there was a steady flow of postdocs through the microwave
lab. These included, in the 1956-1970 period: Robert Kurland, Victor Laurie,
Ted Sarachman, Leonard Nugent, Bill Kirchhoff, Bob Kuczkowski, Ralph Nelson,Wallace
Pringle, Don Johnson, Frank Lovas, and Jean Jacob. Several of these postdocs went
on to become permanent NBS employees. Foreign Guest Scientists included
Takahiro Kasuya and Chi Matsumura from Japan, A. M. Ronn from Israel, and
Daniel Christensen from Denmark. Francis Powell from Catholic University and
Larry Krisher from the University of Maryland, both local institutions,
regularly spent time in the NBS microwave lab over many years. Thus, in spite
of tight budgets and NBS personnel restrictions, the microwave group maintained
a very active research program.
There were also many
interactions with other microwave labs both in the U. S. and abroad.
Interactions with Harvard (E. B. Wilson) were particularly strong, but they
included other places such as the National Research Council of Canada (C. C.
Costain), Columbia (C. H. Townes and B. P. Dailey), Berkeley (R. J. Myers), and
Stanford (Victor Laurie). David Lide spent a sabbatical year in 1959-60 with D.
J. Millen at University College London and Børge Bak at Copenhagen, and
another in 1968 with Paolo Favero at the University of Bologna. Members of the
group made many contributions to meetings and conferences, including the Ohio
State Molecular Spectroscopy Symposium, APS and ACS meetings, the biennial
European Molecular Spectroscopy Symposium, and the 1962 International Symposium on Molecular Structure and Spectroscopy in Tokyo.
Specific Studies from 1954 to the
Early 1970s
As mentioned above, the
first thrust of the research program at NBS was to study molecules with
internal motions that produced low-frequency vibrational modes. These studies
are summarized here.
One-top molecules:
·
Methyl
amine (Lid52,Lid54,Lid57a,Kiv57). The complex inversion-internal rotation
motions were elucidated, and the torsional barrier height and inversion
splitting determined.
·
Propylene
(Lid57b,Lid61) and methylallene (Lid57c). Barrier heights determined.
·
Ethyl
chloride (Lid59), ethyl bromide (Lid59), and ethyl cyanide (Lau59a). Barrier
heights determined.
·
Isoprene
(Lid64). Barrier to CH3 rotation shown to be higher than propylene
and similar molecules.
·
Phosphorus
trifluoride-borane (PF3BH3) (Kuc67). A strong Coriolis
interaction allowed an accurate determination of the barrier height.
·
Trimethylamine-trimethylboron
complex (Lid59b). No evidence of internal rotation, but some structural
information obtained.
Two-top molecules:
·
Propane
(Lid60a). The two CH3 groups were shown to be staggered, with the CH3
axis essentially coincident with the C-C bond. The barrier is high. Propane
was shown to have a very small but non-zero dipole moment (0.084 D).
·
Isobutene
(Lau61a). The equilibrium conformation was determined and the methyl barriers
measured.
Three-top molecules:
·
Trimethylamine
(Lid58), trimethylphosphine (Lid58a), and trimethylarsine (Lid59). The energy
levels associated with the torsional motions were determined and limits placed
on the barrier heights.
·
Isobutane
(Lid58a,Lid60), tertiary butyl fluoride (Lid58a), and tertiary butyl chloride (Lid63a)
. Each CH3 group was found to be hindered by a high barrier, with
smaller interactions between the groups.
Low barrier cases:
·
Methyltrifluoromethylacetylene
(Lid55). Published measurements reinterpreted to show a near-zero barrier.
·
1-Chloro-2-butyne
(Lau59). Found to have nearly free internal rotation; upper limit to barrier
was set.
·
Methylsilylacetylene
(Kir65). Barrier was found to be very small but non-zero.
·
Trifluoromethyl
trifluorosilane (CF3SiF3) (Lid72). The barrier to
internal rotation was found to be fairly low, leading to a torsional frequency in
the far infrared.
Butadiene derivatives:
·
Fluoroprene
(Lid62a) and isoprene (Lid64): The carbon skeleton was found to be planar with
a trans configuration. The barrier to rotation about the C-C bond is
very high, with no indication of a cis isomer.
Other studies:
·
Cyanamide
(Mil62,Lid62)). Equilibrium configuration was found to be pyramidal with a very
low barrier to inversion.
·
Difluoramine
(NF2H) (Lid63). Pyramidal configuration with high barrier to
inversion.
·
Tetrafluorohydrazine
(N2F4) (Lid59c). C2v configuration with very
high barrier to internal rotation and inversion.
·
Propyl
chloride (C3H7Cl) (Sar63). Both trans and gauche
conformers were identified and shown to be very close in energy.
The information on
internal motions in the molecules listed above proved valuable in gaining an
overall picture of hindered internal rotation and in testing various theories
and quantum chemical calculations. Furthermore, these studies produced a large
amount of precise data on interatomic distances and angles, electric dipole
moments, and nuclear quadrupole coupling constants. Other molecules studied in
this period for the purpose of measuring structural parameters, dipole moments,
and quadrupole coupling constants included:
· Vinyl chloride (Kiv60)
|
· Hydrazoic acid (For63)
|
· Vinyl fluoride (Lau61)
|
· Perchloryl fluoride (Lid65a)
|
· Chloroform (Jen62)
|
· Difluoroborane (Kas68)
|
· tert-Butyl acetylene (Nug62)
|
· Trifluoramine oxide (Kir69)
|
· tert-Butyl cyanide (Nug62)
|
· Methyl sulfone (Jac71)
|
· Hexafluoropropene (Jac73)
|
· Methyl sulfonyl fluoride (Jac71)
|
· cis-Difluoroethylene (Lau61)
|
· Ethynydifluoroborane(Laf69,Laf71)
|
· Cyclopentene oxide
(Laf70)
|
· Cyanocyclobutane (Dur73)
|
· Silacyclobutane (Pri71)
|
· 3,6-Dioxabicyclo[3.1.0]hexane (Cre73)
|
· Silacyclopentane (Dur76)
|
|
The second major thrust of
the NBS microwave lab in the 1960-70 period was directed at the detection and
study of molecular species present in highly energetic environments. One
motivation for this was the interest at that time in improving rocket performance,
searching for new approaches to missile defense, and other military/space
program applications. The NBS work involved two techniques, (a) generating
short-lived species by electrical discharges and subsequent chemical reactions,
then flowing them through a waveguide cell, and (b) producing molecules by
vaporizing a solid into an absorption cell maintained at elevated temperature.
Successes were achieved with both techniques.
To pursue the first
approach, a high-power electrical discharge cell was constructed in which atoms
of O, H, etc. could be produced. The gas stream containing these atoms was
mixed with another stream containing appropriate molecules and the reaction
products pumped through a special absorption cell. The cell (Lid64b) was
designed for Stark modulation with a Δm = ±1 selection rule. It
allowed the gas to flow through freely, and the walls could be coated so as to
retard destruction of the transient molecules. This technique was used to study
several transient species:
- Sulfur
monoxide (SO) (Pow64). The spectrum of this free radical, an analog of O2,
is complicated by interaction of the electron spin with the overall rotation.
The observed spectrum was explained satisfactorily and the coupling parameters
measured.
- Hydroxyl
radical (OH) (Pow65). Although the OH spectrum had been previously observed,
the stronger signals obtained with the NBS apparatus allowed the first precise
measurement of the electric dipole moment.
- Difluoromethylene
(CF2) (Pow66,Kir73). A detailed analysis of the spectrum, including
centrifugal distortion effects, led to definitive values of the structure,
dipole moment, and vibrational force field.
Refinements and extensions of this
technique, to be discussed later, produced important data on a wide range of transient
species of interest in radioastronomy and other areas.
The investigations of
molecules present in high-temperature gases employed a similar type of
waveguide cell, this time contained in a quartz jacket inside a furnace. A tray
below the waveguide contained a solid sample of the substance of interest. The
molecules studied included:
- Lithium
chloride (LiCl) (Lid64a). The rotational constants and interatomic distance
were determined with high precision, as well as the variation of dipole moment
with vibrational state and the dipole derivative.
- Aluminum
monofluoride (AlF) (Lid63b,Lid65). This compound was produced by reacting AlF3
with Al in the heated cell. Precise rotational constants, vibration-rotation
interaction constants, quadrupole coupling constant, and electric dipole moment
were obtained. These measurements indicated a highly ionic Al-F bond.
- Aluminum
monochloride (Lid65). Similar measurements were done on AlCl.
- Cesium
hydroxide (CsOH) (Kuc66,Lid67,Lid69) and rubidium hydroxide (RbOH) (Mat69,Lid69).
These spectra were observed in the vapor above the solid compound. Interatomic
distances, dipole moments, and other parameters were measured, and a rough
value of the metal-oxygen stretching frequency was obtained. The molecules were
shown to be linear with a very large amplitude bending vibrational mode, which
leads to unusual patterns of vibrational satellites.
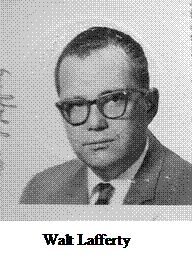
As
a result of
reorganizations at the National Bureau of Standards in the early 1960s,
the
microwave group was merged with the high-resolution infrared group that
had
been built up by Earle K. Plyler. The combined section, called Molecular
Spectroscopy, was led by David Lide. Plyler had constructed what was
probably
the most advanced high-resolution infrared instrument in the world at
that time
and had brought in several young spectroscopists including Arthur Maki,
Walter
Lafferty (shown here), and Bruce Olson. This merger led to highly
productive
symbiosis between the microwave and infrared laboratories. A number of
molecules were investigated in both spectral regions, yielding new
information
on vibration-rotation interactions.
Examples include:
·
HCN
and DCN (Lid67a, Mak67, Mak68, Mak78)
·
FCN (Laf67)
·
ClCN (Laf65)
·
N2O
(Laf64)
·
HCCCN
(Laf67a)
·
CH3CN
(Laf67a)
·
DCCD
(Laf77)
·
OCS
(Mak67a, Mak73)
·
OCSe
(Mak77)
The work on HCN was notable in that
it was used to explain the mechanism of far infrared laser action in HCN, which
had been incorrectly attributed to the CN molecule (Lid67a, Mak67). This work
led to other measurements that shed light on other gas phase laser transitions
such as CS2 (Mak67b) and DCN and other HCN laser transitions (Mak68,
Mak78). The HCN laser played a significant part in the 1972 measurement of the
speed of light to unprecedented accuracy, a measurement that led to a new
definition of the meter as the standard of length.
In the fall of 1967, Don
Johnson, from the C. C. Lin group at the University of Oklahoma, joined the
microwave team at NBS as a Postdoctoral fellow under the guidance of David
Lide. New laboratory space for the Molecular Spectroscopy Group had just been
completed at the Gaithersburg site. Working with Francis X. Powell, a guest
worker from Catholic University, Johnson’s first task was to set the equipment
from the Connecticut and Van Ness site in the new laboratory space in
Gaithersburg to provide for future research. It had been decided that the focus
of much of the future research effort in the new laboratory would be on
developing microwave techniques for studying short lived chemical intermediates
that play important roles in many gas phase reactions. Fortunately, the
Molecular Spectroscopy group had amassed huge collection of spectroscopic
equipment from past experiments. Perhaps the most important to the future
efforts was an amazing collection of reflex klystrons providing tunable
radiation from about 2 GHz to well over 100 GHz.
The technology of
microwave spectroscopy was still at a very primitive stage in 1967 and most of
the apparatus that would be needed had to be designed and built for the task.
Most of the short lived molecules that Powell and Johnson hoped to study had
not been studied in the gas phase before and their microwave spectra could not
be predicted very well. They could anticipate lengthy searches for very weak
signals so the microwave sources they intended to use needed to be stabilized
both electronically and thermally while remaining easy to use on a daily basis.
Commercial electronic stabilization equipment was just being developed at the
time and was too difficult to use for broad searches requiring several
different klystrons. Their solution was very simple. Each klystron was sealed
and submerged in its own 5 gallon container of automotive motor oil and
operated with reduced dc voltage on the filament. They also developed their
own vacuum tube sweep circuits and signal amplifiers in order to keep the
system noise as low as possible.
Applications in Radio Astronomy and
Atmospheric Chemistry
Most of the short lived
molecules that Johnson and Powell hoped to study would be likely to exist only
in such small concentrations that their absorption path length would be a few
centimeters at best. Parallel plate absorption cells were developed for these
studies with Teflon coated plates and very high speed pumping systems to allow
the chemistry to be optimized in electrical discharge production systems. The
free radical, ClO, was the first to be studied with this system followed
quickly by BrO and SF2. In later years ClO and BrO became
quite important
in the atmospheric reaction mechanisms in the destruction of the ozone
layer.
In 1969 the first organic
interstellar molecule was detected by L.E. Snyder, and coworkers (Sny69). This
inspired Johnson to undertake the study of a related molecule, thioformaldehyde
(H2CS) (Joh70) which was detected in interstellar clouds a few years
later towards Sgr B2 at the Parkes 64 m radio telescope in Australia (Sinclair et
al. Aust. J. Phys. 26, 85 (1973)).
In 1970 Frank Lovas
joined the NBS microwave group as a Postdoctoral fellow of David Lide. Since
Lide had left the group in 1969 to direct the Office of Standard Reference
Data, Lovas and Johnson began collaborating on lab studies. Their first joint
study was on the radical, BF, (Lov71) produced in a microwave discharge of BF3.
Later, they studied the transient molecule CH2=NH (Joh72) produced
by fluorine atom abstraction from methyl amine. These experiments employed the
millimeter wave parallel plate cell shown here. CH2NH is
isoelectronic with formaldehyde and thus a potential interstellar molecule. As
in the case of thioformaldehyde, an Australian team using the Parkes 64 m radio
telescope detected the methanimine 110 – 111 transition
with 14N hyperfine structure toward the galactic center cloud Sgr B2
(God73).
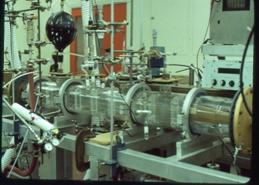
These early laboratory
studies on interstellar molecules pointed to the need of comprehensive
microwave spectral data, i.e. spectral predictions beyond the measured data
sets, so Johnson, Lovas and Kirchhoff initiated the data series “Microwave
Spectra of Molecules of Astrophysical Interest” with the first publication on
Formaldehyde, Formamide and Thioformaldehyde (Joh72a) with predicted spectra up
to 300 GHz which covered the receiver range of radio telescopes at that time. Kirchhoff
had developed the fitting code for other projects but it was ideal for
predicting spectra with firm statistical uncertainties (Kir72).
In 1972 Bill Kirchhoff
left the Molecular Spectroscopy Section and joined the Office of Air and Water
Measurements, thus providing an opening to hire Lovas into the Molecular
Spectroscopy Section. In addition to continuing microwave lab studies, one of
Lovas’ duties was to take over the Molecular Spectroscopy Data Center funded by
the Office of Standard Reference Data. This involved the production of the
Microwave Spectral Tables, the first on Diatomic molecules (Lov74) and
continuing the series on Microwave Spectra of Molecules of Astrophysical
Interest. In 1973-74 Eberhard Tiemann from the Freie Universität Berlin spent
a year sabbatical working with Lovas and Johnson on studies of transient
species such as the SO-dimer, OSSO, (Lov74a) produced in a microwave discharge
of SO2 employing the centimeter wave parallel plate cell shown
here.
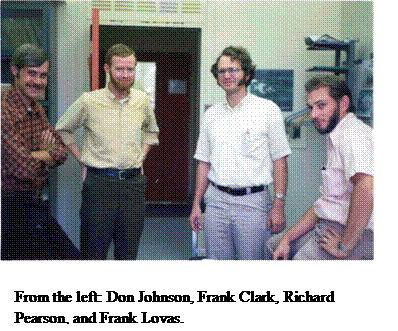
During the early 1970’s
Johnson and Lovas began a long-term collaboration with Lew Snyder and his
students at the University of Illinois with the detection of interstellar
dimethyl ether (Sny74) toward the Orion Nebula. Also in 1973 one of Snyder
students, F. O. Clark joined NBS as a Postdoctoral fellow with Johnson to
carry out both lab and interstellar molecular studies. The first lab study
Clark worked on with Lovas and Tiemann was the pyrolysis of ethylamine to
produce the transient species vinyl amine (Lov75). On the interstellar front,
Johnson, Clark, and Lovas joined a host of other astronomers in the detection
of trans-ethanol toward Sgr B2 (Zuc75). Also in 1975 Johnson and Lovas were
co-authors of a paper describing the detection of interstellar sulfur dioxide,
SO2, with L.E. Snyder as lead author (Sny75).
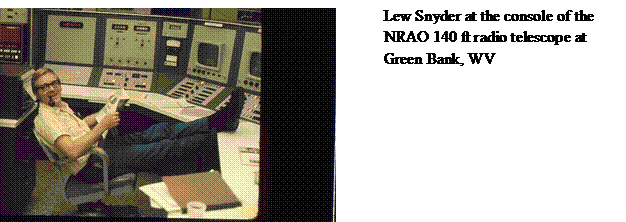
In 1974 Richard Pearson
joined the microwave group as a Postdoctoral fellow with Lovas. Pearson was
involved in a variety of spectral studies, but one important result was the
determination of the structure of CH2NH (Pea77). As opposed to
producing CH2NH with F atom abstraction from methylamine, Lovas and
Pearson employed pyrolysis of methylamine and 13C or 15N
isotopically enriched forms. For the normal species the best production was
found to be from pyrolysis of 1,2-diaminoethane (ethylenediamine) as noted in
the earlier study of vinyl amine (Lov75).
Based on observations of
several Harvard astronomers, which were communicated to Johnson and Lovas,
ethyl cyanide was identified in the Orion Nebula cloud OMC-1 (Joh77) by means
of 24 transitions. The existing laboratory data at that time were measurements
below 41 GHz, while the astronomical data set ranged from 88 GHz to 116 GHz, so
the literature data was supplemented with new measurements in the 89 GHz to 118
GHz range to provide a firmer identification. As a result of their laboratory
and astronomical studies on interstellar molecules, Johnson and Lovas were
awarded the Department of Commerce Gold Medal in 1976, the highest award of the
Department.
In 1975 Rick Suenram
joined the group as a Postdoctoral fellow with Don Johnson with an interest in
atmospheric and interstellar molecules. One of the first lab studies Suenram
worked on with Johnson and Lafferty was the microwave spectrum of cyanamide
(Joh76), which had been detected in the Sgr B2 interstellar cloud by Turner et
al. (Tur75) the previous year. Suenram and Johnson (Sue76, Sue77) also began a
series of studies of the chlorine nitrate molecule (ClONO2), which
was found to be an important atmospheric species in reactions causing the
destruction of the ozone layer. A number of years later, Suenram and Lovas
extended the spectral measurements on chlorine nitrate to the millimeter-wave
range (Sue84) since it was becoming clear that atmospheric monitoring of
chlorine nitrate was essential and this frequency range had higher sensitivity.
With some internal
funding from the Office of Air and Water Measurements, Suenram and Lovas began
work on the ozone-olefin reaction system, which is important in the troposphere
in forming smog. Rick and Frank began a room temperature stopped flow study of
ethylene and ozone reaction products in the parallel plate Stark cell. After
completing a series of experiments, Rick had the idea to freeze out the two
reactants in a stainless steel Stark cell, shown below, by introducing each
separately while the cell was cooled with liquid N2 (preventing any
reaction before warming the cell).
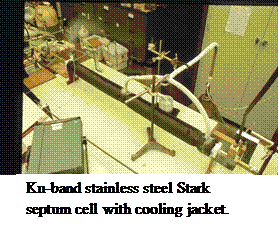
Once the ozone and
ethylene were introduced and frozen out, the cell was slowly warmed and
spectral scans undertaken. Formaldehyde was observed first at a temperature of
-130 °C and then the dioxirane (H2CO2) spectrum appeared
between -100 °C and -84 °C before disappearing. Over the years this reaction
has received considerable attention by organic chemists. The mechanism of
reaction has the ozone terminal oxygen atoms adding across the C=C bond,
forming a primary ozonide five-membered ring, which then cleaves to form
formaldehyde and the H2COO radical (so called Criegee intermediate
(Cri57)) as shown in Stage 1 of the proposed mechanism for reaction pictured on
the next page. Suenram and Lovas found that the H2COO radical is
stabilized by forming the three-membered CO2 ring of dioxirane
before decomposing to the final products CO, H2O, CO2 and
H2 measured by mass spectrometry by NBS scientists Richard Martinez,
John Herron and Robert Huie in a stopped flow study of the ozone-ethylene
reaction.
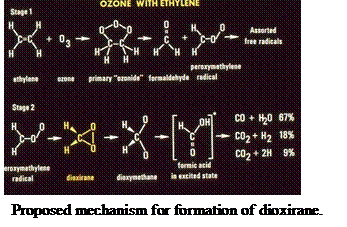
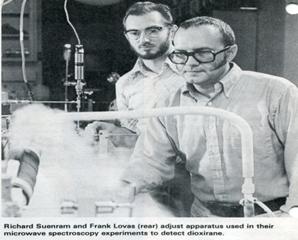
It is well
established that some fraction of the two initial fragments, formaldehyde and H2COO,
recombine to form a secondary ozonide, whereby the five-membered ring has the
sequence _OCOOC_ and this is also observed in the microwave experiment at
temperatures above -80 °C. By employing various isotopic forms of ethylene and
ozone, Suenram and Lovas studied isotopically-labeled dioxirane to obtain its
substitution structure shown here. (Sue78)
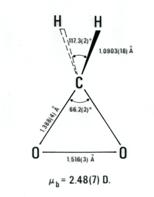
When Rick Suenram began
his postdoctoral studies at NBS, one of his objectives was to obtain the
rotational spectrum of glycine, the simplest amino acid. Once the laboratory
spectrum was observed and assigned it would then be possible to search for it in
the interstellar medium using radio telescopes. Unfortunately most amino acids
exist at room temperature as solid substances with negligible vapor pressure.
In order to get enough substance in the vapor phase for a microwave study, the
amino acid has to be heated. Also by nature of being a biomolecule, amino
acids are somewhat susceptible to thermal decomposition so they are difficult
to vaporize without decomposing them. In order to study them Rick designed and
built a millimeter wave, parallel plate, absorption cell that was capable of
being heated to several hundred degrees Celsius using external heating tapes.
The cell was constructed of Pyrex and gold plated metals to minimize
decomposition. A small quartz boat containing the sample to be vaporized was
placed in the center of the cell, directly beneath the gold plated parallel
plates. Using this cell, the millimeter wave spectrum of glycine was observed
and assigned (Sue78). As with many organic molecules, there are possibilities
for conformational isomerism due to internal hydrogen bonding within the
molecule. Glycine was no exception and the first conformer observed had a
large dipole moment along the a-molecular axis. Subsequent ab-initio
calculations suggested that the experimentally observed conformer was in fact
not the lowest energy conformer and hence might not be the most likely
conformer to be observed in the interstellar medium. (Sch80) Nevertheless,
several interstellar searches were undertaken for this conformer in
collaboration with Lew Snyder and his students. These searches all turned out
to be unsuccessful.
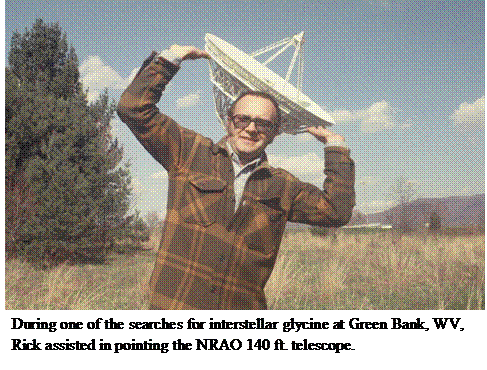
Based on
the results and predictions of Sch80, new laboratory searches were undertaken
for the predicted lowest energy conformer of glycine. After some searching, a
weaker spectrum of a second conformer was observed and assigned (Sue80). The
spectrum of this conformer was weaker due to the fact that the dipole moment
was much smaller than that for the first observed conformer. Based on the
newly assigned spectrum, additional interstellar searches were undertaken but
again they were unsuccessful in detecting glycine in the interstellar medium.
(Hol80).
During the period 1980 to
1981 Bob Kuczkowski made a sabbatical visit to NIST working with Lovas and
Suenram. One of the interesting studies Bob was involved in was the millimeter
wave study of the spectrum of sulfuric acid to obtain its molecular structure.
A parallel plate cell that was originally built to study glycine was used since
it was all glass or gold coated metal and thus not subject to the corrosive
acid. Also, it was designed to be heated to several hundred degrees Celsius by
means of heating tapes. In addition to studying the normal isotopic form at
temperatures near 100 °C, the 34S, d1 and d2
deuterated forms were studied, allowing the structure to be determined (Kuc81).
Lovas and Suenram began
studies on radical and transient species employing the microwave discharge and
millimeter wave parallel plate cell. Using a flowing mixture of H2S
in N2, they generated the NS radical and measured the N = 1-0, 2-1
and 3-2 rotational hyperfine patterns (Lov82) over the frequency range 67 GHz
to 162 GHz and predicted the spectrum up to 300 GHz for radio astronomy
applications. In addition to generating the NS radical, two new species were
also identified: thiohydroxylamine (H2NSH) and sulfur diimide
(HSNSH). Both cis and trans forms of H2NSH were assigned
(Lov83). For HSNSH two of the possible three planar conformers were observed,
the cis-trans and cis-cis (Sue85)
In 1983, with
the assistance of Ken Evenson from the NBS Boulder Lab, Lovas and Suenram began
a search for the NKK = 404 – 313 transition of
the X 3B1 ground state of the CH2 radical
between 68 GHz and 71 GHz based on predictions from the far infrared
measurements from prior Evenson studies. A microwave discharge of F2
in He generated F atoms that were used to extract hydrogen atoms from methane
as shown in the figure to the right. After some searching, three hyperfine
triplets were detected corresponding to the J = 5-4, 4-3 and 3-2 transitions.
This work was reported in the Astrophysical Journal due to its importance in
radio astronomy (Lov83a). While the initial search for interstellar CH2
was only partially successful (Hol89), the detection was later confirmed by
Hollis, Jewell, and Lovas (Hol95) toward the Orion-KL and W51 M molecular
clouds.
In the early 1980’s
Suenram and Lovas turned their attention to studying several species important
in atmospheric chemistry, particularly in the destruction of ozone, with
support from the Chemical Manufacturers Association. The first of these
studies was an extension of measurements on chlorine nitrate into the
millimeter wave range (Sue84). The second species studied was hypochlorous
acid, HOCl, with collaborators from the University of British Columbia (M.C.L.
Gerry’s lab) and the JPL spectroscopists Cohen and Pickett (Sin84). This was
followed with the study of peroxynitric acid (HOONO2) over a
frequency range from 40 GHz to 189 GHz. The spectrum of the ground state of
HOONO2 exhibited tunneling splittings on the order of 5 MHz to 10
MHz due to tunneling of the OH across the heavy atom plane (Sue86).
In the mid-1980’s the Molecular
Spectroscopy Division initiated a new direction which focused on the
spectroscopy (both infrared and microwave) of molecular dimers and clusters.
Suenram and Lovas began their effort with the study of HF dimer (Laf87) and H2CO-HF
(Lov87) employing the stainless-steel Ku band Stark septum cell
previously used in the study of dioxirane. This allowed them to cool the cell
to enhance the formation of dimers. Realizing that this method would not work
for more weakly bound dimers, they began construction of a pulsed molecular
beam Fabry-Perot cavity Fourier Transform Microwave Spectrometer (FTMW) which
had recently been developed by Bill Flygare’s group at the University of
Illinois (commonly known as the Balle-Flygare instrument. (Bal81)).
Fabry-Perot Cavity Fourier Transform
Microwave Spectrometer
It turns out that this
new technique would lead to a worldwide renaissance of rotational spectroscopy
over the next three decades. The initial configuration of the NIST instrument
was described by Lovas and Suenram in a paper on rare gas complexes of OCS
(Lov87a). This version had two microwave oscillators phase locked to each
other with a 30 MHz IF and required manual stepping of the master oscillator
and the Fabry-Perot cavity mirror. Mirror movement was achieved by means of
manually stepping the motor micrometers behind each mirror as illustrated here.
Quite a large
number of rare gas molecular complexes and molecular dimers were studied with
this spectrometer but here we will just highlight the ones of special
interest. At this point in time, the water dimer was of high interest both
experimentally and theoretically due to multiple tunneling motions. Water
dimer has eight equivalent frameworks giving rise to five pairs of
vibration-rotation species: E±, A1±, B1±,
A2±, and B2±. The initial lab
study at NBS was reported in Cou87 and later Coudert and Hougen reported the
theoretical model and a global analysis of all microwave and far infrared data
available (Cou90). This included the data reported by Fraser, Suenram and
Coudert taken with the newly developed microwave electric-resonance optothermal
spectrometer (Fra89).
The ammonia dimer is
another example of a species exhibiting complex tunneling motions. Jerry
Fraser, who came to NBS as a Postdoctoral fellow working with Alan Pine , had
initially studied the ammonia dimer with the molecular beam electric resonance
spectrometer at Harvard with Nelson and Klemperer. Jerry carried out zero
field hyperfine measurements of four isotopic variants of ammonia dimer with
the FTMW spectrometer (Nel87).
During 1987-88 Woody
Gillies and Jennifer Zozom (Gillies) made several extended visits to the NBS
microwave lab. They participated in the FTMW study of the formamide –water and
formamide-methanol complexes (Lov88). Both complexes were found to have two
hydrogen bonds, the oxygen of water (methanol) bound to the H of the HNCO
moiety and the OH hydrogen to the oxygen atom of the HNCO group.
During one of these
visits they also returned to studies of the ozone-ethylene system in an attempt
to produce the primary ozonide of ethylene. This is the five-member ring
structure where the terminal O-atoms of ozone bridge the C–C bond and produces
the initial product in the reaction scheme shown earlier. However, due to its instability,
no one had ever observed it spectroscopically. The same experimental procedure
used in the dioxirane study was followed, except the cell temperature was
maintained near -100 °C for up to 6 hours after warm up from -196 °C by flowing
cooled N2 gas through the cylinder surrounding the waveguide cell.
By this method, the primary ozonide of ethylene was stabilized and its
millimeterwave spectrum was observed (Zoz87). Later, a structural study was
carried out using various isotopic forms enriched in 13C, D, and 18O
(Gil88). A year later, on another visit by the Gillies, the ozone-ethylene van
der Waals complex was studied with the FTMW spectrometer (Gil89). The complex
was found to have the same basic structure as the primary ozonide defining the
reaction coordinate of the 1,3-dipolar cycloaddition leading to the primary
ozonide shown here. A more detailed structural study was reported several
years later (Gil91).
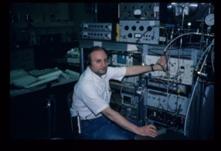
In 1988 Stew Novick
(shown at right) visited NBS on sabbatical from Wesleyan University. His
interest was the structural study of the van der Waals heterodimer OCS-CO2.
It was anticipated that the dimer would have a slipped parallel structure like
the carbon dioxide dimer, and this proved to be the case (Nov88). Later on
Stew duplicated one of the NBS FTMW spectrometers for his use at Wesleyan based
on the drawings provided by Suenram and Lovas. Later the Wesleyan shop
constructed three others for use at Harvard, Mt. Holyoke College and Amherst
College.
About this same time
Helen Leung and Mark Marshall, came to NBS to study the N2-H2O
complex which exhibits the same type of interchange of the donor and acceptor
atoms in the H-bond as does water dimer, however only four equivalent
frameworks exist in this case (Leu89). During 1988 and later Karen Peterson
from the University of Rhode Island came to NBS with an interest in studying
trimers containing water. The first study involved CO2 and H2O
to form the CO2-CO2-H2O complex (Pet89). The
structure found contained the planar CO2 dimer in a slipped parallel
structure with the water above the plane and the water oxygen lone pair
directed at the two carbon atoms. No tunneling was detected. The second study
also involved CO2 and H2O but the trimer complex formed
was H2O-H2O-CO2. The structure found has all
the heavy atoms in a plane and perhaps the hydrogens as well since the dipole
moment in the c-axis direction is zero (Pet91).
In all the studies
mentioned to date, the FTMW instrument was manually scanned and this was a major
impediment to getting things accomplished in a timely fashion. It was time to
move on to the next step in instrument development and improvement. In order
to automate the scans, a number of electronic modifications to the FTMW
instrument were first necessary. A single-sideband modulator (SSBM) was
introduced in the electronics along with a 30 MHz source (tripling the 10 MHz
reference signal), which allows one to remove the second microwave oscillator
by using a power splitter with one output to the SSBM and the second output to
the detector mixer (Sue89). The schematic below shows the layout that was
ultimately used in the development and construction of the portable FTMW
spectrometers at NIST in the mid to late 1990s. (Sue99). With all the improvements
that were made during approximately a decade of experiments, the sensitivity of
the FTMW instrument was now in the ppb range making it viable to observe many
isotopomers in natural abundance.
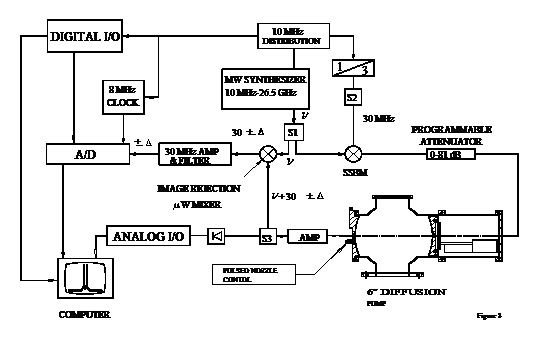
Keiji Matsumura from
Seinan Gakuin University in Japan spent a sabbatical year working with Lovas
and Suenram on laser ablation studies as well as molecular dimers. One of the
dimer studies Keiji carried out was on the deuterated and partially deuterated
acetylene dimer( Mat91) which exhibits interesting tunneling.
As the FTMW technique
began to mature in the late 1980’s and early 1990’s, Suenram and Lovas looked
to ways to extend the technique beyond simple hydrogen bonded species and van der
Waals clusters. One of the most fruitful means was to employ different nozzle
designs. Suenram and Lovas developed a variety of pulsed nozzle
configurations to facilitate the study of other types of molecular compounds
and complexes. Shown here are several of the nozzles designs that they
developed.
The flow nozzle was
employed when the two species whose complex was sought reacted and formed a
solid, e.g. NH3 and HX (X = halogen). The flow nozzle was also used
in the ozone-ethylene experiments to observe the ozone-ethylene van der Waals
complex. (Gil89). During several more visits by Woodie and Jennifer Gillies,
the dual flow nozzle also proved useful in studying ketene-acetylene (Gil93)
and ketene-ethylene (Lov94). These were of interest because Woodward and
Hoffmann suggested that the reaction could be viewed as a concerted (2πs
+ 2πa) cycloaddition with a transition state characterized by
crossed, mutually perpendicular molecular planes of ketene and the alkene. In
the case of ethylene the structure found was close to what Woodward and
Hoffmann suggested, but for acetylene a planar structure was found.
The reservoir
nozzle can be heated routinely up to 200 °C for species with low vapor pressure
at room temperature. It has been employed extensively over the years at NIST
and the design has been reproduced and used at many other microwave labs around
the world. Probably one of the most difficult experiments using this nozzle
involved the study of corannulene (C20H10) where Lovas
and Grabow heated the nozzle to 220 ºC to 250 ºC (Lov05). In the
course of this study, several nozzle coils burned out due to the high
temperature employed. Corannulene was of interest since it is polar, as
opposed to many of the polycyclic aromatic hydrocarbons (PAHs) which are
non-polar and have no microwave spectrum. The PAHs are thought to be the
carriers of the interstellar diffuse bands.
The DC discharge nozzle was employed in the study
of radicals or transient interstellar species to measure low-J rotational
transitions and to determine the dipole moments (Lov92, Sue94). The laser
ablation pulsed nozzle source was used to study refractory materials or other
solids with low vapor pressure. Some of the first studies involved SiC2
(Sue89) and the refractory metal oxides YO, LaO, ZrO, and HfO (Sue90). Later
this technique was used for microwave measurements on BaO and SrO and combined
with new infrared measurements on SrO and earlier infrared measurements to give
improved Dunham potential constants for BaO and SrO (Blu92). These experiments
were the first applications coupling laser vaporization to an FTMW instrument.
It also showed that the FTMW instrument had the sensitivity to observe refractory
compounds. Note that the oxides of Y, La, Zr and HF were previously only seen
using optical absorption experiments. The diagram here shows the basic
configuration employed.
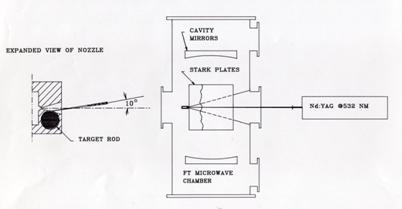
In the early 1990s the
long-standing relationship with the Russian millimeter wave group headed by A.
F. Krupnov was greatly strengthened. This was made possible in part by the
lifting of the iron curtain. Rick made two trips to Nizhny Novgorod (formerly
Gorky) in the early 1990s to work firsthand with many members of Krupnov’s
group. (Bel92) Prior to this, Nizhny Novgorod was a closed city, inaccessible
to Westerners. In this same timeframe, NIST also hosted a number of Krupnov’s
staff where Lovas and Suenram worked on many projects of mutual interest both
in the conventional millimeter frequency region and the FTMW region.
Among those that visited NIST were S.
P. Belov, M. Yu. Tretyakov, and E. N. Karyakin. Belov and Tretyakov worked
with Lovas and Suenram on the study of several molecular complexes, namely CH3OH-CO
(Lov94a) and methanol dimer (Lov95). One of the interesting results of these
studies was that the internal rotation barrier of the CH3 rotor was
substantially lowered, a factor of two or more compared to free methanol. Another
interesting aspect of the methanol dimer study was that 16 transitions occur
for each of the K = 0, a-type rotational transitions. One of the early studies
that Karyakin also carried out was the donor-acceptor tunneling in HDO-DOH and
HDO-HOD (Kar95).
Of particular note is the
work with Andrey Zuban and Igor Leonov on the automation of the FTMW
spectrometers at NIST. Andrey and Igor were hardware and software specialists,
respectively, that had more than a thorough understanding of using computers to
automate instrumentation. Together they developed a software package known as
LZ98 that was used to control FTMW spectrometers, not only at NIST but also at
other labs in the US, Europe, and Japan. (Sue99) This software permitted long
(many GHz), unattended searches to be carried out with this type of
spectrometer thus making it possible to set up a particular set of chemistry
conditions and blindly search to see what was present in the chemical system being
studied without having any preconceived notions of where to look for a
particular species. It cannot be emphasized strongly enough how important this
was in every type of system studied by FTMW instruments all the way from large
organic compounds where multiple conformers are present [1-octene, 15
conformers (Fra01)] to small metal oxides produced by laser vaporization [ZrO2,
Bru99, HfO2 (Les02)]. In the metal oxide experiments, a number of
strong transitions were observed in the several GHz that were scanned. It
turned out that these transitions belonged to the metal dioxides, which had
never been seen previously in the gas phase.
Suenram and Lovas entered
a major long-term collaboration with the rotational spectroscopist, Jens-Uwe
Grabow, from Kiel, Germany. In the mid 1990’s Jens began the first of several
extended visits to NIST as a Postdoctoral fellow. One of the most noteworthy
studies Jens carried out at NIST was on the very weakly bound rare gas dimer
Ne-Ar which required a 1 Watt amplifier to boost the microwave input power to
the cavity (Gra95). Jens and Yoshi Kawashima, another frequent visitor to the
NIST lab, also worked on the spectrum of conformers I and II of glycine to
determine the dipole moments and resolve the 14N hyperfine structure.
In these studies, glycine was produced both in a heated nozzle and by laser
vaporization (Lov95a). Jens was also principal author on the FTMW study of N2O5
which exhibited large amplitude motions from the two NO2 groups
which undergo internal rotation tunneling via a geared rotation of the two
units about their C2 axes (Gra96).
Jens also developed an
automated software package for control of the FTMW type instruments but one of
the main collaborations at NIST was working with Suenram on the development of
a scaled down (smaller) version of the FTMW instrument that was totally
transportable. (Sue99). In the late 1990’s, several of these spectrometers
were constructed. One of these instruments was taken to Aberdeen, Maryland and
installed in an Army surety laboratory and used to observe and analyze the
rotational spectra of several of the nerve agents for the first time, first
Sarin (Wal01), and then Soman (Sue04). This instrument and one of the sister
instruments at NIST were also used to study a large number of agent related
compounds from 2000 to 2005 with support from the Army Research Office.
(DaBel04, Sue04).
Another study by Suenram
and coworkers was on dimethyl methylphosphonate which is among the organo-phosphorus
compounds which are relatively harmless but serve as model compounds in place
of nerve agents. The spectrum showed an unexpected complication in that the
methoxy groups tunneled to produce equivalent structures in addition to the
internal rotation of the three methyl groups (Sue02). In the initial report
only the A-state was fit, but later a global analysis was carried out (Oha03).
In the mid 1990s, David
Plusquellic was hired at NIST. David’s area of expertise is high resolution
UV, visible laser spectroscopy but he has had a major impact in microwave
spectral analysis. During his tenure as a graduate student at David Pratt’s
laboratory at the University of Pittsburgh he began the development of a
Graphical User Interface (GUI) program to simulate optical spectra. The
program is known as JB95 and is available from NIST at http://www.nist.gov/pml/div682/grp01/jb95.cfm
One advantage of this program was that it utilized the ground state rotational
constants to simulate rotational spectra. One could overlay a theoretical
spectrum from assumed or ab initio calculated rotational constants on
the laboratory scan. The assumed rotational constants, dipole moment
components, and resulting spectra are all computer mouse controlled by track
bars. This permits rotational assignments to be varied “on the fly” to see if
a match can be obtained to the observed spectrum (spectra). Once a close match
or pattern is observed, the transitions can be assigned in a “point and click”
fashion using a computer mouse and trial fits obtained. This literally reduced
assignment times to sometimes a few minutes for spectra that might otherwise
have taken days or weeks to analyze. Using this program, one can simulate up
to nine separate spectra at once. Also, once a particular spectrum (conformer)
has been assigned, it can be digitally subtracted from the overall spectrum to
simplify the remaining spectra. This is true not only for multiple conformers,
but isotopomers as well (13C species for instance). Needless to
say, it would have been difficult if not impossible to study and assign the
spectra of quite a few of the larger molecules that we have studied without the
use of this program, e.g. see 1-pentene (Fra00), 1-octene (Fra01), and
1-heptanal (Fis06) for instance.
In early 2000 Lovas’
interest returned to interstellar molecules. In May 2000 Hollis, Lovas and
Jewell used the NRAO 12 m radio telescope near Tucson, AZ (shown here) to study
the simplest sugar, glycolaldehyde. They detected 6 transitions in the galactic
center cloud Sgr B2(N) at frequencies between 71 GHz and 104 GHz (Hol00). This
was the last time the group used the 12 m, since in June 2000 NRAO turned over
the operation of the instrument to the Astronomy Department of the University
of Arizona.
In a later study of
interstellar glycolaldehyde with the BIMA array telescope, Hollis et al.
(Hol01) showed that the spatial extent exceeded 60" rather than being
confined to the hot core (about 5" in diameter) of the cloud Sgr
B2(N-LMH). From the same data taken in May 2000, Hollis and co-workers
identified ethylene glycol (antifreeze), which is the sugar alcohol of
glycolaldehyde (Hol02). Shortly after the glycolaldehyde detection, Lovas
spent six months at the Astronomy Department of the University of Illinois
working with Lew Snyder and his students.
Meanwhile at NIST,
Fraser, Suenram and a visiting scientist Cathrine Lugez embarked upon a series
of studies involving 1-alkenes which were known to have large numbers of
molecular conformers in room temperature gas phase samples. It was surmised
that in a molecular beam, a much simpler picture would emerge as all the higher
energy conformers would cool down to the lowest energy conformer and only the
spectrum of the lowest energy conformer would be seen in the molecular beam.
The authors were surprised by the results in that the spectrum that was
observed was quite complex, indicating that a number of conformers were present
in the molecular beam. While it is true in some cases that conformers will freeze
out, if there are barriers to internal rotation that inhibit a higher energy
conformer from getting to a lower energy conformer, the individual conformers
will remain suspended in the gas phase and emerge from the nozzle unscathed.
(While the alkanes were of primary interest, they tend to have very small
dipole moments and thus are not amenable to microwave studies.) The C=C double
bond in the alkenes assures that all have a dipole moment. The first study
reported on seven conformational isomers of the expected 13 for 1-hexene
(Fra00). This was followed with the observation of four of the five expected
conformers of 1-pentene (Fra00a). A year later these authors reported the
observation of fifteen conformers of 1-octene (Fra01).
While the study of molecular
complexes was waning at NIST, the ozone-methane complex drew the interest of
Angela Hight Walker and coworkers since it was involved in the study of the O +
CH4 reaction as a means to fix the time zero in measuring the
product appearance in this fast reaction. Thus the geometry and internal
dynamics of this complex was of interest. The FTMW study of the O3-CH4
complex showed that the CH4 undergoes nearly free internal rotation
which leads to A, E, and F internal-rotation sublevels which correlate to those
three rotational states in free methane (Wal00).
Rich Lavrich joined the
group as a postdoctoral fellow from 2001 to 2003. He initially worked with
Suenram, but when Suenram retired Lavrich was supervised by David Plusquellic.
Together they carried out a number of studies on conformational analysis of
peptide mimetics. The first of these was on the alanine dipeptide analog N-acetyl-alanine
N'-methylamide (Lav03). With three methyl rotors, 14 torsional states
are expected. However only three of these were analyzed, the AA, AE and EA
states which accounted for the vast majority of the spectral features
observed. A second study Rich carried out was on the peptide mimetic
ethylacetamidoacetate (also known as N-acetylglycine ethyl ester) that yielded
two low energy conformers (Lav03a).
The Green Bank Telescope
(GBT) was found to be very good for the detection of larger organic molecules.
Lovas and co-workers identified cyanoallene toward the TMC-1 molecular cloud
aided by its hyperfine structure (Lov06). This detection was followed by the
detection of a new ring molecule, cyclopropentone (c-H2C3O)
also with the 100 m GBT(Hol06). The authors suggested a formation route for
cyclopropenone via the addition of an oxygen atom to cyclopropenylidine (c-C3H2)
as shown here:
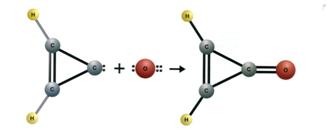
Shortly after this
methyltriacetylene (CH3C6H) was detected toward TMC-1
(Rem06). This is the largest symmetric top molecule detected to date. Working
from GBT data collected in 2004 and 2005, Hollis and coworkers identified
interstellar acetamide (CH3C(=O)NH2) toward Sgr B2(N-LMH)
by means of 7 A and E state rotational transitions (Hol06a). This is the
largest interstellar molecule with a peptide linkage, i.e. the HNCO backbone.
These workers reported the detection of keteneimine (CH2=C=NH)
toward the hot core of Sgr B2(N) which is a higher energy isomer of methyl
cyanide (Lov06a).
The last two parts of the
series "Microwave
Spectra of Molecules of Astrophysical Interest" were published in 2007 and
2008. These were Part XXV on methylamine (Ily07) and Part XXVI on acetic acid
(Ily08), two important interstellar species exhibiting internal rotation. In
March of 2008 Remijan and coworkers reported the detection of cyanoformaldehyde
toward Sgr B2(N) with the GBT (Rem08). The formation mechanism is postulated
to be from a radical neutral molecule reaction of CN with H2CO
yielding CNCHO + H. The team of Hollis, Lovas, Jewell and Remijan detected 8
new interstellar species with the GBT over a two year period.
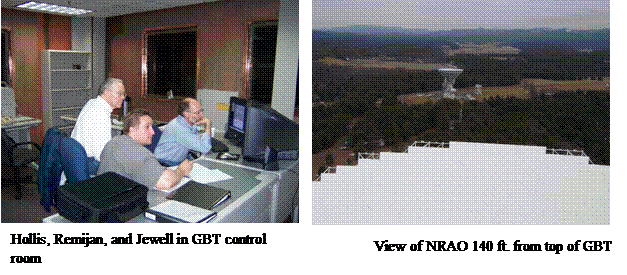
Returning to laboratory
studies, several interesting polyols with three carbon atoms were studied as
potential interstellar molecules. The first of these was glycerol, CH2OHCHOHCH2OH,
which has 12 conformational isomers. From the FTMW spectral scans 5
conformers were identified with the aid of ab initio calculations. One
of these showed an interesting tunneling between its two chiral forms
(Ily08a). The second study was on 1,2-propanediol. It is an asymmetric top molecule
with at least 23 low energy conformers. Its spectrum was scanned with the NIST
cavity FTMW spectrometer as well as the broadband chirped-pulsed FTMW
spectrometer in Brooks Pate’s lab at the University of Virginia. The seven
lowest energy conformers were assigned with the aid of ab initio
calculations. The two lowest energy forms were sought unsuccessfully with the
GBT toward Sgr B2(N-LMH) (Lov08). We thought this might be successful since it
only differs from the known interstellar molecule ethylene glycol by an
additional methyl group. The final study of these C3 polyols was on
1,3-propanediol. As in the previous case both cavity and broadband FTMW
spectrometers were used. Ab initio calculations were carried out on the
8 lowest energy forms to aid the spectral analysis. In this case only the two
lowest energy conformers were assigned and both exhibited tunneling between
their two chiral forms (mirror images).
Both Frank Lovas and Rick
Suenram formally retired from NIST in 2000 but remained as guest scientists for
a number of years. At the moment only Lovas remains in the microwave lab, so
before long this story will end for the microwave lab. However, Dave
Plusquellic and Kevin Douglass have recently developed a chirped-pulse
broadband terahertz spectrometer in the region (500 GHz– 600 GHz) and
demonstrated its sensitivity for trace gas sensing (Ger11). Thus, future
rotational spectroscopy at NIST will likely move to their laboratory.
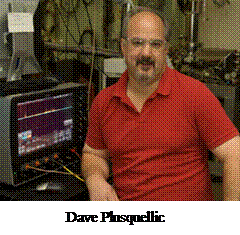 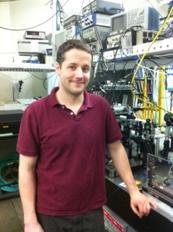
In the references below
and cited in the text, nearly all of the lab studies reported up to about 1970
are given. After 1970, because of space limitations, we have only described
roughly 25 % of those published during this very active period. For a more complete
list the reader is encouraged to search the Web of Science (or similar
databases) for publications from individual staff members and their visitors.
References
App06 A.J. Apponi, D.T.
Halfen, L.M. Ziurys, J.M. Hollis, A.J. Remijan, and F.J. Lovas, Astrophys. J.
(Letters) 643, L29 (2006), "Investigating the Limits of Chemical
Complexity in Sgr B2(N): A Rigorous Attempt to Confirm
1,3-Dihydroxyacetone".
Bal81 T.J. Balle and
W.H. Flygare, Rev. Sci. Instrum. 52, 33 (1981), "Fabry-Perot Cavity
Pulsed Fourier Transform Microwave Spectrometer with a Pulsed Nozzle Particle
Source" .
Bel92 S.P. Belov, M.Yu
Tretyakov, and R.D. Suenram, Astrophys. J. 393, 848 (1992),
"Improved Laboratory Rest Frequency Measurements and Pressure Shift and
Broadening Parameters for the J=2¬1 and J=3¬2 Rotational Transitions of CO".
Blu92 C. E. Blum, H. G.
Heddrich, F. J. Lovas, R. D. Suenram, and A. G. Maki, J. Mol. Spectrosc. 152,
109 (1992), "Infrared and Microwave Spectrum of SrO and BaO".
Bru99 D.J. Brugh, R.D.
Suenram, and W.J. Stevens, J. Chem. Phys. 111, 3526 (1999),
"Fourier Transform Microwave Spectroscopy of Jet-cooled ZrO2 Produced
by Laser Vaporization".
Cou87 L.H. Coudert,
F.J. Lovas, R.D. Suenram, and J.T. Hougen, J. Chem. Phys. 87, 6290
(1987), "New Measurements of Microwave Transitions in the Water
Dimer".
Cou90 L.H. Coudert and
J.T. Hougen, J. Mol. Spectrosc. 139, 259 (1990), "Analysis of the
Microwave and Far Infrared Spectrum of the Water Dimer".
Cre73 R.A. Creswell and W.J. Lafferty, J.
Mol. Spectrosc. 46, 371 (1973), "Microwave Spectrum, Dipole Moment,
and Conformation of 3,6‑Dioxabicyclo [3. 1.0] Hexane".
Cri57 R. Criegee,
Records Chem. Prog. 18, 11 (1957) .
DaB04 R.S. DaBell, R.D.
Suenram, R.J. Lavrich, J. Michael Lochner, M.W. Ellzy, Kenneth Sumpter, J.O.
Jensen, and A.C. Samuels, J. Mol. Spectrosc., 228, 230 (2004), "The
Geometry of Organophosphonates: Fourier-Transform Microwave Spectroscopy and ab
initio study of Diethyl Methylphosphonate, Diethyl Ethylphosphonate, and
Diisopropyl methylphosphonate ".
Dur73 J.R. Durig, L.A. Carreira, and W.J.
Lafferty, J. Mol. Spectrosc. 46, 187 (1973), "Spectra and Structure of Small Ring
Compounds: Microwave Spectrum of Cyanocyclobutane".
Dur76 J.R. Durig, W.J. Lafferty and V.F.
Kalanisnsky, J. Phys. Chem. 80, 1190 (1976) ,"Spectra and Structure
of Small-Ring Molecules: 33. Microwave spectrum of Silacyclopentane".
Fiz06 J. M. Fisher, L. H.
Xu, R. D. Suenram, B. H. Pate, and K. O. Douglass, J. Mol. Struct. 795,
143 (2006), "Conformational Isomerism in 1-Heptanal".
For63 R.A. Forman and
D.R. Lide, Jr., J. Chem. Phys. 39, 1133 (1963), "Quadrupole
Coupling Constants from the Microwave Spectrum of Hydrazoic Acid".
Fra89 G.T. Fraser, R.D.
Suenram, and L.H. Coudert, J. Chem. Phys. 90, 6077 (1989),
"Microwave Electric-Resonance Optothermal Spectroscopy of (H2O)2".
Fra00 G.T. Fraser,
R.D. Suenram, and C.L. Lugez, J. Phys. Chem. A 104, 1141 (2000),
"Rotational Spectra of Seven Conformational Isomers of 1-Hexene".
Fra00a G.T. Fraser,L.H.
Xu, R.D. Suenram, and C.L. Lugez, J. Chem. Phys. 112, 6209 (2000),
"Rotational Spectra of Four Conformers of 1-Pentene".
Fra01 G.T. Fraser,
R.D. Suenram, and C.L. Lugez, J. Phys. Chem. A 105, 9859 (2001), "Investigation
of Conformationally rich molecules: Rotational Spectra of Fifteen
Conformational Isomers of 1-Octene".
Ger11 E. Gerecht, K.O.
Douglass, and D.F. Plusquellic, Optics Express 19 (9), 8973 (2011),
"Chirped-pulse Terahertz Spectroscopy for Broadband Trace Gas
Sensing".
Gil88 J.Z. Gillies,
C.W. Gillies, R.D. Suenram, and F.J. Lovas, J. Am. Chem. Soc. 110, 7991
(1988), "The Ozonolysis of Ethylene. Microwave Spectrum, Molecular Structure
and Dipole Moment of the Primary Ozonide (1,2,3-Trioxolane)".
Gil89 J.Z. Gillies,
C.W. Gillies, R.D. Suenram, F.J. Lovas, and W. Stahl, J. Amer. Chem. Soc. 111,
3073 (1989), "The Microwave Spectrum and Molecular Structure of the
Ethylene-Ozone van der Waals Complex".
Gil91 C.W. Gillies and
J.Z. Gillies, R.D. Suenram, F.J. Lovas, E. Kraka, and D. Cremer, J. Am. Chem.
Soc. 113, 2412 (1991), "Van der Waals Complexes in 1,3-Dipolar
Cycloaddition Reactions: Ozone-ethylene".
Gil93 C.W. Gillies,
J.Z. Gillies, F.J. Lovas, and R.D. Suenram, J. Am. Chem. Soc. 115, 9253
(1993), "The Rotational Spectrum and Structure of a Weakly Bound Complex
of Ketene and Acetylene".
God73 P.D. Godfrey, R.D.
Brown, B.J. Robinson, and M.W. Sinclair, Astrophys. Lett. 13, 119 (1973)
"Discovery of Interstellar Methanimine (Formaldimine) ".
Goo46 W.E. Good, Phys. Rev.
69, 539 (1946), "The Inversion Spectrum of Ammonia".
Gra95 J.-U. Grabow,
A.S. Pine, G.T. Fraser, F.J. Lovas, R.D. Suenram, T. Emilsson, E. Arunan, and
H.S. Gutowsky, J. Chem. Phys. 102, 1181 (1995), "Rotational Spectra
and van der Waals Potentials of Ne-Ar".
Gra96 J.-U. Grabow,
A.M. Anders, G.T. Fraser, K.K. Irikura, R.D. Suenram, F.J. Lovas, W.J.
Lafferty, and J.L. Domenech, J. Chem. Phys. 105, 7249 (1996),
"Microwave Spectrum, Large-amplitude Motions, and Ab Initio Calculations
for N2O5".
Hol80 J.M. Hollis, L.E.
Snyder, R.D. Suenram, and F.J. Lovas, Astrophys. J. 241, 1001 (1980),
"A Search for the Lowest - Energy Conformer of Interstellar Glycine".
Hol89 J.M. Hollis, P.R.
Jewell, and F.J. Lovas, Astrophys. J. 346, 794 (1989), "A Search
for Methylene in the Orion Nebula".
Hol95 J.M. Hollis, P.R.
Jewell, and F.J. Lovas, Astrophys. J. 438, 259-264 (1995),
"Confirmation of Interstellar Methylene".
Hol00 J.M. Hollis, F.J.
Lovas, and P.R. Jewell, Astrophys. J. (Letters) 540, L107 (2000),
"Interstellar Glycolaldehyde: The First Sugar".
Hol01 J.M. Hollis, S.N.
Vogel, L.E. Snyder, P.R. Jewell, and F.J. Lovas, Astrophys. J. (Letters) 554,
L81 (2001), "The Spatial Scale of Glycolaldehyde in the Galactic
Center".
Hol04 J.M. Hollis, P.R.
Jewell, F.J. Lovas, A. Remijan, and H. Møllendal, Astrophys. J. (Letter)
610, L21 (2004), "Green Bank Telescope Detection of New
Interstellar Aldehydes: Propenal and Propanal".
Hol06 J.M. Hollis, A.J.
Remijan, P.R. Jewell, and F.J. Lovas, Astrophys. J. 642, 933 (2006),
"Cyclopropenone (c-H2C3O): A New Interstellar Ring
Molecule".
Hol06a J.M. Hollis, F.J.
Lovas, A.J. Remijan, P.R. Jewell, V.V. Ilyushin, and I. Kleiner, Astrophys. J.
(Letters) 643, L25 (2006), "Detection of Acetamide (CH3CONH2):
The Largest Interstellar Molecule with a Peptide Bond".
Ily07 V. Ilyushin and
F.J. Lovas, J. Phys. Chem. Ref. Data 36 (3), 1141 (2007),
"Microwave Spectra of Molecules of Astrophysical Interest. XXV.
Methylamine".
Ily08 V. Ilyushin, I.
Kleiner, and F.J. Lovas, J. Phys. Chem. Ref. Data 37 (1), 97 (2008),
"Microwave Spectra of Molecules of Astrophysical Interest. XXVI. Acetic
Acid".
Ily08a V.V. Ilyushin,
R.A. Motiyenko, F.J. Lovas, and D.F. Plusquellic, J. Mol. Spectrosc. 251,
129 (2008), "Microwave Spectrum of Glycerol: Observation of a Tunneling
Chiral Isomer".
Jac71 E.J. Jacob and D.R. Lide, Jr., J. Chem. Phys. 54, 4591 (1971),
"Microwave Investigation of Methyl Sulfone and Methane Sulfonyl
Fluoride".
Jac73 E.J. Jacob and D.R. Lide, Jr., J. Chem. Phys. 59, 5877 (1973),
"Structural Implications of the Microwave Spectrum of
Hexafluoropropene".
Jen62 M. Jen and D.R.
Lide, Jr., J. Chem. Phys. 36, 2525 (1962), "Molecular Structure of
Chloroform".
Joh70 D.R. Johnson and
F.X. Powell, Science 169, 679 (1970), "Microwave Detection of
Thioformaldehyde".
Joh72 D.R. Johnson and
F.J. Lovas, Chem. Phys. Lett. 15, 65 (1972), "Microwave Detection
of the Molecular Transient Methyleneimine (CH2=NH)".
Joh72a D.R. Johnson,
F.J. Lovas, and W.H. Kirchhoff, J. Phys. Chem. Ref. Data 1, 1011 (1972),
"Microwave Spectra of Molecules of Astrophysical Interest I.
Formaldehyde, Formamide, and Thioformaldehyde".
Joh76 D.R. Johnson,
R.D. Suenram, and W.J. Lafferty, Astrophys. J. 208, 245 (1976),
"Laboratory Microwave Spectrum of Cyanamide".
Joh77 D.R. Johnson,
F.J. Lovas, C.A.Gottlieb, E.W. Gottlieb, M.M. Litvak, M. Guelin, and P.
Thaddeus, Astrophys. J. 218, 370 (1977), "Detection of Interstellar
Ethyl Cyanide".
Kar95 E.N. Karyakin,
G.T. Fraser, F.J. Lovas, R.D. Suenram, and M. Fujitake, J. Chem. Phys. 102,
1114 (1995), "Donor-Acceptor Interchange Tunneling in HDO-DOH and the
Higher Energy HDO-HOD Isotopomer".
Kas68 T. Kasuya, W.J.
Lafferty, and D.R. Lide, J. Chem. Phys. 48, 1 (1968), "Microwave
Spectrum, Structure, Boron Quadrupole Coupling Constants, and Dipole Moment of
Difluoroborane".
Kir65 W.H. Kirchhoff
and D.R. Lide, Jr., J. Chem. Phys. 43, 2203 (1965), "Microwave
Spectrum and Barrier to Internal Rotation in Methylsilylacetylene".
Kir69 W.H. Kirchhoff
and D.R. Lide, Jr., J. Chem. Phys. 51, 467 (1969), "Microwave
Spectrum, Dipole Moment, and Quadrupole Coupling Constant of Trifluoramine
Oxide".
Kir72 W.H. Kirchhoff, J.
Mol. Spectrosc. 41, 333 (1972), "On the Calculation and
Interpretation of Centrifugal Distortion Constants: A Statistical Basis for
Model Testing: The Calculation of the Force Field ".
Kir73 W.H. Kirchhoff,
D.R. Lide, and F.X. Powell, J. Mol. Spectrosc. 47, 491 (1973), "The
Microwave Spectrum, Force Field and Dipole Moment of CF2".
Kiv57 D. Kivelson and
D.R. Lide, Jr., J. Chem. Phys. 27, 353 (1957), "Structure of the
Methylamine Molecule. II. Theory of Internal Motions and Application to CD3ND2
".
Kiv60 D. Kivelson, E.B.
Wilson, Jr., and D.R. Lide, Jr., J. Chem. Phys. 32, 205 (1960), "Microwave
Spectrum, Structure, Dipole Moment, and Nuclear Quadrupole Effects in Vinyl
Chloride".
Kuc81 R.L. Kuczkowski,
R.D. Suenram, and F.J. Lovas, J. Am. Chem. Soc. 103, 2561 (1981)
"The Microwave Spectrum, Structure and Dipole Moment of Sulfuric
Acid".
Kuc66 R.L. Kuczkowski,
D.R. Lide, Jr., and L.C. Krisher, J. Chem. Phys. 44, 3131 (1966),
"Microwave Spectra of Alkali Hydroxides: Evidence for Linearity of CsOH
and KOH".
Kuc67 R.L. Kuczkowski
and D.R. Lide, Jr., J. Chem. Phys. 46, 357 (1967), "Microwave
Spectrum, Structure, Dipole Moment, and Barrier to Internal Rotation of
Phosphorus Trifluoride---Borane".
Laf64 W.J. Lafferty
and D.R. Lide, J. Mol. Spectrosc. 14, 407 (1964), "Rotational Constants of
Excited Vibrational States of 14N216O".
Laf65 W.J. Lafferty,
D.R. Lide, and R.A. Toth, J. Chem. Phys. 43, 2063 (1965), "Infrared
and Microwave Spectra of ClCN".
Laf67 W.J. Lafferty
and D. R. Lide, J. Mol. Spectrosc. 23, 94 (1967), "Microwave
Spectrum of Excited Vibrational States of FCN".
Laf67a W.J. Lafferty, J.
Mol. Spectrosc. 25, 359 (1967) , "Direct l‑type
Doubling Transitions in Some Axially Symmetric Molecules".
Laf69 W.J. Lafferty
and J.J. Ritter, Chem. Comm. 909, 1969, "Microwave Spectrum, Structure,
and Dipole Moment of Ethynyldifluoroborane, HCCBF2".
Laf70 W.J. Lafferty,
J. Mol. Spectrosc. 36, 84 (1970), "Microwave Spectrum, Dipole
Moment, and Conformation of Cyclopentene Oxide".
Laf71 W.J. Lafferty,
and J.J. Ritter, J. Mol. Spectrosc. 38, 181 (1971), "Microwave
Spectrum, Dipole Moment, and Structure of Ethynyldifluoroborane".
Laf77 W.J. Lafferty,
R.D. Suenram and D.R. Johnson. J. Mol. Spectrosc. 64, 147 (1977),
"Microwave Spectrum of Acetylene-d2".
Laf87 W.J. Lafferty,
R.D. Suenram, and F.J. Lovas, J. Mol. Spectrosc. 123, 434 (1987),
"Microwave Spectra of the (HF)2, (DF)2, HFDF, and
DFHF Hydrogen-Bonded Complexes ".
Lau59 V.W. Laurie and
D.R. Lide, Jr., J. Chem. Phys. 31, 939 (1959), "Microwave Spectrum
and Internal Rotation of 1-Chloro-2-Butyne".
Lau59 V.W. Laurie, J.
Chem. Phys. 31, 1500 (1959), "Microwave Spectrum and Internal Rotation
of Ethyl Cyanide".
Lau61 V.W. Laurie, J.
Chem. Phys. 34, 291 (1961), "Microwave Spectrum of cis-Difluoroethylene.
Structures and Dipole Moments of Fluoroethylenes".
Lau61a V.W. Laurie, J.
Chem. Phys. 34, 1516 (1961), "The Microwave Spectrum of Isobutylene. Dipole
Moment, Internal Barrier, Equilibrium Conformation, and Structure".
Lav03 R.J. Lavrich,
D.F. Plusquellic, R.D. Suenram, G.T. Fraser, A.R. Hight Walker, and M.J.
Tubergen, J. Chem. Phys. 118, 1253 (2003), "Experimental Studies of
Peptide Bonds: Identification of the C7eq Conformation of
the Alanine Dipeptide Analog N-Acetyl-alanine N'-Methylamide from
Torsion-rotation Interactions ".
Lav03a R.J. Lavrich,
A.R. Hight Walker, D.F. Plusquellic, I. Kleiner, R.D. Suenram, J.T. Hougen, and
G.T. Fraser, J. Chem. Phys. 119, 5497 (2003), "Conformational
Analysis of the Jet-cooled Peptide Mimetic Ethylacetamidoacetate from
Torsion-rotation Spectra".
Leu89 H.O. Leung, M.D.
Marshall, R.D. Suenram, and F.J. Lovas, J. Chem. Phys. 90, 700 (1989),
"Microwave Spectrum and Molecular Structure of the N2-H2O
Complex".
Les02 A. Lesarri, R.D.
Suenram, and D.J. Brugh, J. Chem. Phys., 117, 9651 (2002),
"Rotational Spectrum of Jet-Cooled HfO2 and HfO".
Lid50 D. R. Lide and
D. Coles, Phys. Rev. 80, 911 (1950), "Microwave Spectroscopic
Evidence for Internal Rotation in Methyl Silane".
Lid52 D.R. Lide, Jr.,
J. Chem. Phys. 20, 1812 (1952), "Preliminary Analysis of the Pure-Rotational
Spectrum of Methyl Amine".
Lid54 D. R. Lide, J.
Chem. Phys. 22, 1613 (1954), "Effects of Internal Motion in the
Microwave Spectrum of Methyl Amine".
Lid55 D.R. Lide, Jr.
and D. Kivelson, J. Chem. Phys. 23, 2191 (1955), "Internal Rotation
in Methyl-Trifluoromethyl Acetylene".
Lid57 D.R. Lide, Jr.,
D.E. Mann, and R.M. Fristrom, J. Chem. Phys. 26, 734 (1957),
"Microwave Spectrum and Structure of Sulfuryl Fluoride ".
Lid57a D.R. Lide, Jr.,
J. Chem. Phys. 27, 343 (1957), "Structure of the Methylamine
Molecule. I. Microwave Spectrum of CD3ND2 ".
Lid57b D.R. Lide, Jr.
and D. E. Mann, J. Chem. Phys. 27, 868 (1957), " Microwave Spectra
of Molecules Exhibiting Internal Rotation. I. Propylene ".
Lid57c D.R. Lide, Jr.
and D. E. Mann, J. Chem. Phys. 27, 874 (1957), " Microwave Spectra
of Molecules Exhibiting Internal Rotation. II. Methylallene ".
Lid58 D.R. Lide, Jr.
and D. E. Mann, J. Chem. Phys. 28, 572 (1958), " Microwave Spectra
of Molecules Exhibiting Internal Rotation. III. Trimethylamine ".
Lid58a D.R. Lide, Jr.
and D. E. Mann, J. Chem. Phys. 29, 914 (1958), " Microwave Spectra
of Molecules Exhibiting Internal Rotation. IV. Isobutane, Tertiary Butyl
Fluoride, and Trimethylphospine ".
Lid58 D.R. Lide, Jr.,
J. Chem. Phys. 30, 37 (1959), " Microwave Spectra of Molecules
Exhibiting Internal Rotation. V. Barrier Height in Ethyl Chloride and Ethyl
Bromide ".
Lid59 D. R. Lide, Jr.,
Spectrochim. Acta 15, 473 (1959), "Microwave Spectrum of
Trimethylarsine".
Lid59a D.R. Lide, Jr.,
R.W. Taft, Jr., and P. Love, J. Chem. Phys. 31, 561 (1959),
"Microwave Absorption in the Trimethylamine-Trimethylboron Addition
Complex".
Lid59a D.R. Lide, Jr.
and D. E. Mann, J. Chem. Phys. 31, 1129 (1959), "Microwave Spectrum
and Structure of N2F4 ".
Lid60 D.R. Lide, Jr.,
J. Chem. Phys. 33, 1519 (1960), "Structure of the Isobutane
Molecule; Change of Dipole Moment on Isotopic Substitution ".
Lid60a D.R. Lide, Jr.,
J. Chem. Phys. 33, 1514 (1960), "Microwave Spectrum, Structure, and
Dipole Moment of Propane".
Lid61 D.R. Lide, Jr.
and D. Christensen, J. Chem. Phys. 35, 1374 (1961), "Molecular
Structure of Propylene".
Lid62 D.R. Lide, J. Mol. Spectrosc. 8, 142 (1962),
"Vibration-Rotation Interactions in Cyanamide; The Question of Planarity
of Amides".
Lid62a D.R. Lide, Jr.,
J. Chem. Phys. 37, 2074 (1962), "Microwave Studies of Butadiene
Derivatives. I. Spectrum of Fluoroprene".
Lid63 D.R. Lide, Jr.,
J. Chem. Phys. 38, 456 (1963), "Microwave Spectrum and Structure of
Difluoramine".
Lid63a D.R. Lide, Jr.
and M. Jen, J. Chem. Phys. 38, 1504 (1963), "Microwave Spectrum of
Tertiary Butyl Chloride. A Comparison of Tertiary Butyl Structures".
Lid63b D.R. Lide, Jr.,
J. Chem. Phys. 38, 2027 (1963), "Microwave Spectrum of Aluminum
Monofluoride".
Lid64 D.R. Lide, Jr.
and M. Jen, J. Chem. Phys. 40, 252 (1964), "Microwave Studies of
Butadiene Derivatives. II. Isoprene".
Lid64a D.R. Lide, Jr.,
P. Cahill, and L.P. Gold, J. Chem. Phys. 40, 156 (1964), "Microwave
Spectrum of Lithium Chloride".
Lid64b D.R. Lide, Jr.,
Rev. Sci. Instrum. 35, 1226 (1964), "Versatile Stark Waveguide for
Microwave Spectroscopy".
Lid65 D.R. Lide, Jr.,
J. Chem. Phys. 42, 1013 (1965), "High-Temperature Microwave
Spectroscopy : AlF and AlCl".
Lid65a D.R. Lide, Jr.,
J. Chem. Phys. 43, 3767 (1965), "Microwave Spectrum of
Perchlorylfluoride".
Lid67 D.R. Lide, Jr. and R.L.
Kuczkowski, J. Chem. Phys. 46, 4768 (1967), "Structure of the Alkali
Hydroxides. I. Microwave Spectrum of Gaseous CsOH".
Lid67a D.R. Lide, Jr., and
A.G. Maki, Appl. Phys. Letters 11, 62 (1967), "On the Explanation
of the So-Called CN Laser".
Lid69 D.R. Lide, Jr.
and C. Matsumura, J. Chem. Phys. 50, 3080 (1969), "Structure of the
Alkali Hydroxides. IV. Interpretation of Vibration–Rotation Interactions in
CsOH and RbOH and Refinement of Structures".
Lid72 D.R. Lide, Jr., D.R.
Johnson, K.G. Sharp, and T.D. Coyle, J. Chem. Phys. 57, 3699 (1972),
"Microwave Spectrum and Barrier to Internal Rotation in CF3SiF3".
Lov71 F.J. Lovas and D.
R. Johnson, J. Chem. Phys. 55, 41 (1971), "Microwave Spectrum of
BF".
Lov74 F.J. Lovas and E.
Tiemann, J. Phys. Chem. Ref. Data 3, 609 (1974), "Microwave
Spectral Tables. I. Diatomic Molecules".
Lov74a F.J. Lovas, E.
Tiemann, and D.R. Johnson, J. Chem. Phys. 60, 5005 (1974),
"Spectroscopic Studies of the SO2 Discharge System. II.
Microwave Spectrum of the SO Dimer".
Lov75 F.J. Lovas, F.O.
Clark, and E. Tiemann, J. Chem. Phys. 62, 1925 (1975), "Pyrolysis
of Ethylamine. I. Microwave Spectrum and Molecular Constants of
Vinylamine".
Lov77 F.J. Lovas and
R.D. Suenram, Chem. Phys. Lett. 51, 453 (1977), "Identification of
Dioxirane (H2COO) in Ozone-Olefin Reactions via Microwave
Spectroscopy".
Lov82 F.J. Lovas and
R.D. Suenram, J. Mol. Spectrosc. 93, 416 (1982), "Reaction Products
from a Microwave Discharge in N2 and H2S. I. The
Microwave Spectrum of NS".
Lov83 F.J. Lovas, R.D.
Suenram and W.J. Stevens, J. Mol. Spectrosc. 100, 316 (1983),
"Reaction Products from a Discharge of N2 and H2S.
The Microwave Spectrum of H2NSH".
Lov83a F.J. Lovas, R.D.
Suenram, and K.M. Evenson, Astrophys. J. (Letters) 267, Ll31 (1983),
"Laboratory Measurement of the 404 - 313 70 GHz
Transition of Ground State Methylene (CH2)".
Lov87 F.J. Lovas, R.D.
Suenram, S. Ross, and M. Klobukowski, J. Mol. Spectrosc. 123, 167
(1987), "Rotational, Structural, Ab Initio and Semirigid Bender Analysis
of the Millimeter Wave Spectrum of H2CO-HF".
Lov87a F.J. Lovas and R.D.
Suenram, J. Chem. Phys. 87, 2010 (1987), "Pulsed Beam Fourier
Transform Microwave Measurements on OCS and Rare Gas Complexes of OCS with Ne,
Ar, and Kr,".
Lov88 F.J. Lovas, R.D.
Suenram, G.T. Fraser, C.W. Gillies and J. Zozom, J. Chem. Phys. 88, 722
(1988), "The Microwave Spectrum of Formamide-Water and Formamide-Methanol
Complexes".
Lov92 F.J. Lovas, R.D.
Suenram, T. Ogata, and S. Yamamoto, Astrophys. J. 399, 325 (1992),
"Microwave Spectra and Electric Dipole Moments for Low-J Levels of
Interstellar Radicals: SO, C2S, C3S, c-HC3, CH2CC,
and c-C3H2,".
Lov94 F.J. Lovas, R.D.
Suenram, C. W. Gillies, J.Z. Gillies, P.W. Fowler, and Z. Kisiel, J. Am. Chem.
Soc. 116,5285 (1994), "The Microwave Spectrum, Structure, and
Internal Motions of Ketene-Ethylene Complex".
Lov94a F.J. Lovas, S.P.
Belov, M.Yu. Tretyakov, J. Ortigoso, and R.D. Suenram, J. Mol. Spectrosc. 167,
191 (1994), "The Microwave Spectrum and Structure of the CH3OH-CO
Dimer".
Lov95 F.J. Lovas, S.P.
Belov, M.Yu. Tretyakov, W. Stahl, and R.D. Suenram, J. Mol. Spectrosc. 170,
487 (1995), "The a-Type K = 0 Microwave Spectrum of the Methanol
Dimer".
Lov95a F.J. Lovas, Y.
Kawashima, J.-U. Grabow, R.D. Suenram, G.T. Fraser, and E. Hirota, Astrophys.
J. (Letters) 455, L201 (1995), "Microwave Spectra, Hyperfine
Structure, and Electric Dipole Moments for Conformers I and II of
Glycine".
Lov03 F.J. Lovas, R.D.
Suenram, D.F. Plusquellic, and H. Møllendal, J. Mol. Spectrosc. 222,
263 (2003), "The Microwave Spectrum of the C3 Sugars:
Glyceraldehyde and 1,3-Dihydroxy-2-propanone and the Dehydration Product
2-Hydroxy-2-propen-1-al,".
Lov05 F.J. Lovas, R.J.
McMahon, J.-U. Grabow, M. Schnell, J. Mack, L.T. Scott, and R.L. Kuczkowski, J.
Am. Chem. Soc. 127, 4345 (2005), "Interstellar Chemistry: A
Strategy for Detecting Polycyclic Aromatic Hydrocarbons by Radio
Astronomy".
Lov06 F.J. Lovas, A.J.
Remijan, J.M. Hollis, P.R. Jewell, and L.E. Snyder, Astrophys. J. (Letter) 637,
L37 (2006), "Hyperfine Structure Identification of Interstellar
Cyanoallene toward TMC-1".
Lov06a F.J. Lovas, J.M.
Hollis, A.J. Remijan, and P.R. Jewell, Astrophys. J. (Letters) 645,
L137-L140 (2006), "Detection of Ketenimine (CH2CNH) in Sgr
B2(N) Hot Cores".
Lov09 F.J. Lovas, D.F.
Plusquellic, B.H. Pate, J.L. Neill, M.T. Muckle, and A.J. Remijan, J. Mol.
Spectrosc. 257, 82 (2009), "Microwave spectrum of
1,2-propanediol".Mat91 K. Matsumura, F.J. Lovas, and R.D. Suenram, J. Mol.
Spectrosc. 150, 576 (1991), "Fourier-Transform Microwave
Spectroscopy of the Deuterated-Acetylene Dimers: The Interconversion Tunneling
Motions of (DCCD)2, (DCCH)2, DCCD‑DCCH, DCCH‑DCCD,
and HCCH‑DCCH".
Mak67 A.G. Maki and D.R.
Lide, Jr., J. Chem. Phys. 47, 3206 (1967), "Microwave and Infrared
Measurements on HCN and DCN: Observations on l-Type Resonance
Doublets".
Mak67a A. G. Maki, J. Mol. Spectrosc. 23, 110
(1967), "Measurements of the Direct l-Doublet Transitions in Carbonyl
Sulfide".
Mak67b A. G. Maki, Appl.
Phys. Letters 11, 204 (1967), "Interpretation of the CS2
Laser Transitions".
Mak68 A. G. Maki, Appl.
Phys. Letters 12, 122 (1968), "Assignment of Some DCN and HCN Laser
Lines".
Mak73 A. G. Maki and D.
R. Johnson, J. Mol. Spectrosc. 47, 226 (1973), "Ground State and
Vibrationally Excited 16O13C32S, 18O12C32S,
and Other Isotopic Species".
Mak77 A. G. Maki, R. L.
Sams, and R. Pearson, Jr., J. Mol. Spectrosc. 64, 452 (1977),
"Microwave and Infrared Spectra of Isotopically Substituted Carbonyl
Selenide (OCSe)".
Mak78 A. G. Maki, J.
Appl. Phys. 49, 7 (1978), "Further Assignments for the Far-Infrared
Laser Transitions of HCN and HC15N".
Mat69 C. Matsumura and D.R.
Lide, Jr., J. Chem. Phys. 50, 71 (1969), "Structure of the Alkali
Hydroxides. III. Microwave Spectra of RbOH and RbOD".
Mil62 D.J. Millen, G.
Topping, and D.R. Lide, J. Mol. Spectrosc. 8, 153 (1962), "Microwave Spectrum and Nonplanarity
of Cyanamide".
Nel87 D.D. Nelson, W.
Klemperer, G.T. Fraser, F.J. Lovas, and R.D. Suenram, J. Chem. Phys. 87, 6364 (1987), "Ammonia Dimer: Further
Spectral Studies".
Nov88 S.E. Novick, R.D.
Suenram, and F.J. Lovas, J. Chem. Phys. 88, 687 (1988), "Determination
of the Structure of OCS-CO2".
Nug62 L.J. Nugent, D.E.
Mann, and D.R. Lide, Jr., J. Chem. Phys. 36, 965 (1962), "Microwave
Structure Determinations on Tertiary Butyl Acetylene and Tertiary Butyl
Cyanide".
Oha03 N. Ohashi, J.
Pyka, G.Yu. Gollubiatnikov, J.T. Hougen, R.D. Suenram, F.J. Lovas, A. Lesarri,
and Y. Kawashima, J. Mol. Spectrosc. 218, 114 (2003), "Line
Assignments and Global Analysis of the Tunneling-rotational Microwave
Absorption Spectrum of Dimethyl Methylphosphonate".
Pea77 R. Pearson, Jr.
and F.J. Lovas, J Chem. Phys. 66, 4149 (1977), "Microwave Spectrum
and Molecular Structure of Methylenimine".
Pet89 K.I. Peterson,
R.D. Suenram, and F.J. Lovas, J. Chem. Phys. 90, 5964 (1989), "The
Structure of the CO2-CO2-H20 van der Waals
Complex Determined by Microwave Spectroscopy". Erratum: J. Chem. Phys. 92,
5166 (1990).
Pet91 K.I. Peterson,
R.D. Suenram, and F.J. Lovas, J. Chem. Phys. 94, 106 (1991),
"Hydration of Carbon Dioxide: The Structure of H2O-H2O-CO2
from Microwave Spectroscopy".
Plu09 D.F.
Plusquellic, F.J. Lovas, B.H. Pate, J.L. Neill, M.T. Muckle, and A.J. Remijan,
J. Phys. Chem. 113, 12911 (2009), "Distinguishing tunneling
pathways for two chiral conformer pairs of 1,3-propanediol from the microwave
spectrum,".
Pow64 F.X. Powell and
D.R. Lide, Jr., J. Chem. Phys. 41, 1413 (1964), "Microwave Spectrum
of the SO Radical".
Pow65 F.X. Powell and
D.R. Lide, Jr., J. Chem. Phys. 42, 4201 (1965), " Improved
Measurement of the Electric-Dipole Moment of the Hydroxyl Radical".
Pow66 F.X. Powell and
D.R. Lide, Jr., J. Chem. Phys. 45, 1067 (1966), "Microwave Spectrum
of CF2".
Pri71 W.C. Pringle, J.
Chem. Phys. 54, 4979 (1971), "Microwave Spectrum,
Vibration-Rotation Interaction, and Ring Puckering Vibration in Silacyclobutane
and Silacyclobutane-1,1d2".
Rem06 A. Remijan, J.M.
Hollis, L.E. Snyder, P.R. Jewell, and F.J. Lovas, Astrophys. J. (Letters) 643,
L37 (2006), "Methyltriacetylene (CH3C6H) Toward
TMC-1: The Largest Detected Symmetric Top".
Rem08 A.J. Remijan, J.M.
Hollis, F.J. Lovas, W.D. Stork, P.R. Jewell, and D.S. Meier, Astrophys. J
(Letters) 675, L85 (2008), "Detection of Interstellar
Cyanoformaldehyde (CNCHO)".
Sar63 T.N. Sarachman, J. Chem. Phys. 39,
469 (1963), "Microwave Spectrum of Normal Propyl Chloride".
Sin84 H. Gillis
Singbeil , W.D. Anderson, R. Wellington Davis, M.C.L. Gerry, E.A. Cohen, H.M.
Pickett, F.J. Lovas, and R.D. Suenram, J. Mol. Spectrosc. 103, 466‑485
(1984), "The Microwave and Millimeter Wave Spectra of Hypochlorous
Acid".
Sny69 L.E. Snyder, D.
Buhl, B. Zuckerman and P. Palmer, Phys. Rev. Lett. 22, 679 (1969)
"Microwave Detection of Interstellar Formaldehyde".
Sny74 L.E. Snyder, D.
Buhl, P.R. Schwartz, F.O. Clark, D.R. Johnson, F.J. Lovas, and P.T. Giguere,
Astrophys. J. (Lett.) 191, L79 (1974), "Radio Detection of
Interstellar Dimethyl Ether".
Sny75 L.E. Snyder,
J.M. Hollis, B.L. Ulich, F.J. Lovas, D.R. Johnson, and D. Buhl, Astrophys. J.
(Lett.) 198, L81 (1975), "Radio Detection of Interstellar Sulfur
Dioxide".
Sue76 R.D. Suenram,
D.R. Johnson, L.C. Glasgow, and P.Z. Meakin, Geophys. Res. Lett. 3, 611
(1976) "Laboratory Microwave Spectrum of ClONO2 and evidence
for existence of ClONO".
Sue77 R.D. Suenram and
D.R. Johnson, J. Mol. Spectrosc. 65, 239 (1977) "Microwave Spectrum
of Chlorine Nitrate (ClNO3) ".
Sue78 R.D. Suenram and
F.J. Lovas, J. Am. Chem. Soc. 100, 5117 (1978), "Dioxirane. Its
Synthesis, Microwave Spectrum, Structure and Dipole Moment".
Sue78a R.D. Suenram and
F.J. Lovas, J. Mol. Spectrosc. 72, 372 (1978), "Millimeter Wave
Spectrum of Glycine".
Sue80 R.D. Suenram and
F.J. Lovas, J. Am. Chem. Soc. 102, 7180 (1980), "Millimeter
Spectrum of Glycine. A New Conformer".
Sue84 R.D. Suenram and
F.J. Lovas, J. Mol. Spectrosc. 105, 351 (1984), "Millimeter Wave
Spectrum of Chlorine Nitrate".
Sue85 R.D. Suenram,
F.J. Lovas, and W.J. Stevens, J. Mol. Spectrosc. 112, 482 (1985),
"Reaction Products from a Microwave Discharge of N2 and H2S.
The Microwave Spectrum of Sulfur Diimide (H-N=S=N-H)".
Sue86 R.D. Suenram,
F.J. Lovas, and H.M. Pickett, J. Mol. Spectrosc. 116, 406 (1986),
"Millimeter Wave Spectrum and Molecular Conformation of Peroxynitric Acid
(HOONO2)".
Sue89 R. D. Suenram,
F.J. Lovas, G.T. Fraser, J.Z. Gillies, C.W. Gillies, and M. Onda, J. Mol.
Spectrosc. 137, 127 (1989), "Microwave Spectrum, Structure, and
Electric Dipole Moment of Ar-CH3OH".
Sue90 R.D. Suenram,
F.J. Lovas, G.T. Fraser, and K. Matsumura, J. Chem. Phys. 92, 4724
(1990), "Pulsed-Nozzle Fourier-Transform Microwave Spectroscopy of
Laser-Vaporized Metal Oxides: Rotational Spectra and Electric Dipole Moments
of YO, LaO, ZrO, and HfO".
Sue91 R.D. Suenram,
G.T. Fraser, F.J. Lovas, and C.W. Gillies, J. Mol. Spectrosc. 148, 114
(1991), "Microwave Spectra and Electric Dipole Moments of X4Σ-1/2
VO and NbO".
Sue94 R.D. Suenram, and
F.J. Lovas, Astrophys. J. (Lett.) 429, L89 (1994), "Electric Dipole
Moment of C3S".
Sue99 R.D. Suenram, J.-U. Grabow, A. Zuban, and I. Leonov, Rev.
Sci. Instrum. 70 2127 (1999), "A portable, pulsed-molecular beam,
Fourier-transform microwave spectrometer designed for chemical analysis".
Sue01 R.D. Suenram,
F.J. Lovas, D.F. Plusquellic, A. Lesaarri, Y. Kawashima, J.O. Jensen, and A.C.
Samuels, J. Mol. Spectrosc. 211 110 (2001), "Fourier Transform
Microwave Spectrum and Ab Initio Study of
Dimethyl-methylphosphonate".
Sue04 R.D. Suenram, R.S. DaBell, A.R. Hight Walker, R.J. Lavrich,
D.F. Plusquellic, M.W. Ellzy, J.M. Lochner, L. Cash, J.O. Jensen, A.C. Samuels,
J. Mol. Spectrosc. 224, 176 (2004), "The Rotational Spectra of the
Diasteromers of Soman"..
Tur75 B.E. Turner, A.G.
Kislyakov, H.S. Liszt, and N. Kaifu, Astrophys. J. (Lett.) 201, L149
(1975) "Microwave Detection of Interstellar Cyanamide".
Wal00 A.R. Hight Walker,
G.T. Fraser, R.D. Suenram, and F.J. Lovas, J. Chem. Phys. 113, 2139
(2000), "The Structure of O3--CH4 and Implications
for the O + CH4 Precursor-Limited Reaction".
Wal01 A.R. Hight Walker,
R.D. Suenram, A.C. Samuels, J.O. Jensen, M.W. Ellzy, J.M. Lochner, and D.
Zeroka, J. Mol. Spectrosc., 207, 77 (2001), "Rotational Spectrum of
Sarin".
Zoz87 J. Zozom, C.W.
Gillies, R.D. Suenram, and F.J. Lovas, Chem. Phys. Lett. 140, 64 (1987),
"Microwave Detection of the Primary Ozonide of Ethylene in the Gas
Phase".
Zuc75 B. Zuckerman,
B.E. Turner, D.R. Johnson, F.O. Clark, F.J. Lovas, N. Fourikis, P. Palmer, M.
Morris, A.E. Lilley, J.A. Ball, C.A. Gottlieb, M.M. Litvak, and H. Penfield,
Astrophys. J. (Lett) 196, L99 (1975), "Detection of Interstellar
Trans-Ethyl Alcohol".
|